Coevolution is the phenomena where interactions between two species fosters reciprocal evolutionary adaptations. The evolution of these adaptations must display specificity and reciprocity. This means both traits should evolve simultaneously as a product of each other. As such, coevolution can be induced both by agonistic interactions, such as those between predator-prey, or through mutualism, particularly pollinator-host relationships. The diversification of numerous taxa, particularly that of angiosperms and hexapods, can be attributed to coevolution (Kitchell, 1983). Who knew such simple interactions are so vital for biodiversity?
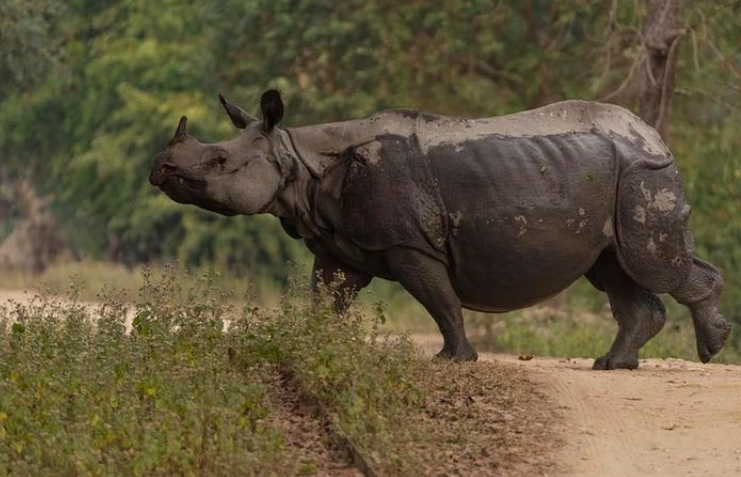
The idea that co-evolution was major driving force of evolution was first suggested by Charles Darwin in 1859 in his famous publication “The Origins of Species”. This was based on his observations between long-spurred flowers and long tongue pollinators (Darwin, 1862). In later publications, Darwin elaborated upon how there were strong evolutionary pressures for spurs longer than the tongue of their pollinators.
The pollinating insect will be required to utilize their entire proboscis to obtain nectar hidden in the plant. This increases the contact of the heads of insects with the reproductive parts of flowers, hence resulting in more extensive pollination (Darwin, 1862). To allow for greater access of nectar, there would be selection favouring longer tongues in the insects, resulting in a spiralling coevolutionary cycle (Darwin, 1862).
Pollinator-Host Coevolution
A prominent example of the pollinator- plant coevolution are the interactions between Large Hawkmoths (Agrius convolvuli) and its plant host Gladiolus longicollis. It was observed that flowers with tube length shorter than that of the tongue of A. convolvuli were not effectively pollinated. Moreover, A. convolvuli was the primary pollinator. Other species possessed significantly smaller tongues and rarely were observed foraging for the nectar of G. longicollis.
Longer tongues has been noted to reduce the maneuverability of bees between flowers. This hence increases the energetic costs of foraging (Harder, 1983). However, the ability to obtain greater amounts of nectar from long-tubed flowers negate the negative effect of long tongues. This a prominent example of how the fitness benefits acquired by co-evolved adaptations often outweigh the associated costs.
Such scenarios emphasise the magnitude at which interspecies interactors influence the evolution of certain adaptations. Despite the costs of the adaptation, reciprocal evolution occurs in response to changing interspecies dynamics. This often leads to a never ending co-evolutionary cycle, which is often associated with exaggerated diversification of certain taxa. In fact, the immense diversity of angiosperms is often attributed to their interactions with aphids.
Diversification of Plants and Aphids occurred Simultaneously
Eudicots form up to 75% of the 352,000 species of angiosperm. Aphids are the predominant pollinators of eudicots. Angiosperm-aphid interactions are generally mutualistic, with the latter relying on their plant host for nectar and floral oils.
As such, aphid crown groups are thought to have originated around 123 Ma. This coincides with the first appearance of the tricolpate pollen. Occurring approximately 125 Ma, this is often acknowledged as the origin of eudicots. It is during this period that the extensive Mid Cretaceous diversification of aphids began (Magallón, 2010).
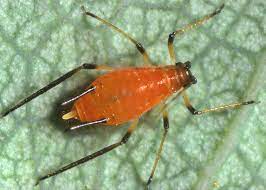
Specialization of Pollination Adaptations
During this period an increase in the specialization of pollination modes amongst angiosperms was noted. Furthermore, phylogenetic studies found that 37% of the extant, zoophilous pollinated, basal angiosperms possessed adaptations for specialized pollinators. Meanwhile 39% had adaptations for generalist insect pollinators. (Hu et al., 2008).
Another study found that traits associated with biotic pollination, namely zygomorphy, resulted in the most profound increase angiosperm species richness (Vamosi and Vamosi, 2010). This is probably because zygomorphic flowers are associated with more specialized pollinators compared to actinomorphic flowers (Yoder et al., 2020) It is for these factors that that aphid pollination is thought to be the impetus for the diversification of angiosperms (Cardinal and Danforth, 2013).
Aphids are also highly specialized Pollinators
The evolution of specialized pollination adaptations is not limited to angiosperms. Aphids too, evolved highly specialized adaptations to allow for efficient nectar foraging. For example, the early spring flower, Lonicera gracilipes, despite being visited by multiple pollinators, has a distinct exclusive mutualistic relationship with A. lonicerae (Shimizu et al., 2014). The mouthparts of other pollinators are not long enough to access the nectar at the base of the base of the corolla tube of L. gracilipes. This implies highly specialized mutualistic relationship between the insect and host plant has been catalysed by rampant coevolution. (Shimizu et al., 2014).
This is supported by Phylogenetic evidence. Out of approximately 1,500 of the Andrena genus, only four species, including A. lonicerae, do not have short tongues. This emphasis the occurrence of co-evolution (Laroca et al., 2022). Nonetheless, specialized aphid-angiosperm relationships exemplify how co-evolution leads to rampant diversification of taxa.
It must be noted however, that without opposing selective forces often leads to highly exaggerated traits. An example of this is the 30-cm long spur of a Malagasy orchid and the tongue of a hawkmoth (Xanthopan morgani praedicta). However, more evidence is required to establish the role of coevolution in the extremity of these traits (Anderson and Johnson, 2007).
Plant- Animal Interactions have led to an Evolutionary Arms Race
The role of co-evolution in the diversification of host-pollinator systems has been well established. However, co-evolution also a major role in agonistic interactions between two species. Exaggerated evolutionary arms race has been observed in parasitic and predator-prey interactions. This is particularly prevalent in relationships between herbivorous insects and their host plant. (Anderson and Johnson, 2007).
Herbivory has instigated Plant-Animal Coevolution
Herbivores have evolved numerous offensive adaptations for efficient foraging of plants. As such, herbivory hinders the both the survival and reproductive success of an individual plant, and hence species. For these reasons, plants evolve defensive mechanism in the form of toxic defence chemicals , mechanical strategies and mimicry.
Subsequently, there will be further radiation of herbivore offensive adaptions in attempt to negate the reciprocal defensive adaptations in plants. This “escape and radiation” coevolutionary mechanism has been credited for the prevalent diversification observed in herbivore-plant systems (Ehrlich and Raven, 1964). A prominent example of this is the evolution of plant chemical defences in response to insect herbivory
Chemical defences are a ubiquitous antiherbivore strategy utilized by a variety of flora. These organic compounds are secondary metabolites which are not primarily involved in either the reproduction or the development of the plant. In fact, the appearance of such compounds, which are not associated with essential photosynthetic and metabolic activity, is thought to have resulted in the evolution of chemical defenses in plants. Chemical defenses can reduce herbivory pressures by either herbivore deterrence or by attracting the predator of the herbivore.
HPIVs
The production of Herbivore Induced Plant Volatiles restricts the damaged associated with herbivory by attracting the predators of the concerned herbivores. An example of this strategy is the production of branched-chain aliphatic acids by the O-acyl sugars of Nictonia attenuate leaves. These compounds attract omnivorous ants which predate on the Manduca sexta larvae. This negates the herbivory pressure instilled by the latter on N. attenuate.
Similarly, the plant, Lotus japonicus, emit volatile compounds, such as germacrene d, 1-octen-3-ol and methyl salicylate (MeSA), amongst others, when infested with herbivorous spider mites (Tetranychus urticae). These compounds would attract the predatory mites (Phytoseiulus persimilis) hence reducing herbivorous pressures. Other plants displaying such chemical defense include corn (Zea mays) (Turlings et al., 1990) and cotton (Gossypium hirsutum) (Dicke et al., 1990).
Often plant chemical defences can induce both physiological damage and depredation of their respective herbivore. For example, the production of quisqualic acid by the petals of the geranium plant, which paralyzes its herbivore, the Japanese beetle (Popillia japonica) following its ingestion. This increases the chances of depredation significantly (Ranger et al., 2011). It must be noted that insects often develop adaptations to counter the chemical defensive mechanisms of plants. (Mithöfer and Boland, 2012).
Herbivores have adapted to these Chemical Defenses
Herbivores often possess a variety of enzymes which are used to detoxify plant chemical defences through oxidation, reduction, hydrolysis or molecular conjecutation (Mithöfer and Boland, 2012). Examples of resistance towards plant chemical defences include the diversification of P450 monooxygenases in the black swallowtail (Papillio polyxenes). This allows the detoxification of furanocoumarins, a toxin produced by its host plant as a defence against herbivory (Scott and Wen, 2001). Similarly, Cabbage white (Pieris rapae) (Wittstock et al., 2004)and diamondback moth (Plutella yxlostella) (Ratzka et al., 2002)utilize their gut proteins to detoxify chemical defensives of their host plant, such as glucosinolate and myosinase. (Ratzka et al., 2002)
Coevolution galore!
The possibility of an evolutionary arms race between herbivorous insects and their host plants was highlighted by a phylogenetic study of the volatile chemistry of 70 species of the tropic genus Bursera . A positive evolutionary trend was observed in incremental chemical diversity and complexity. Newer species possessed a more elaborate suite of chemical defences. The ever-evolving nature of chemical defences proved to be a hindrance for the insect herbivores of these plants. These results reiterate the influence of the “escape and radiation” mechanisms prevalent across coevolved species (Becerra et al., 2009).
Moreover, the evolutionary arms race between herbivorous insects and plants is not limited to the latter’s chemical defence. In fact, positive correlations between the divergence of antiherbivore traits, including mechanical, biotic and chemical strategies, in plant communities and herbivore insect diversity has been observed globally (Becerra, 2015). Furthermore, it was found that specialist herbivores promoted plant species richness more than their generalist counterparts (Becerra, 2015).
Generalist Species are more likely to undergo Coevolution
Constant herbivore pressure will result in the production of novel defenses in the plants to which local herbivores are not adapted to. The evolution of plant defenses will not significant impact Generalist herbivores, such as mammals. This is because of their ability to consume a wider range of plant hosts.
Comparatively, specialist species have significant genetic and physiological constraints. These limit their ability to circumvent the evolution of plant defenses With a reduced diversity of available hosts, specialists undergo strong selection pressures to develop more specialized adaptation to exploit their hosts (Becerra, 2015).
Higher Chemical Diversity Correlates with Butterfly Diversity
As such the composition of plant chemical defences has been observed to significantly influence the diversity the assemblages of butterflies. This was observed during a study of butterfly host interactions amongst four families, namely, Lycaenidae, Pieridae, Nymphalidae, and Hesperiidae.
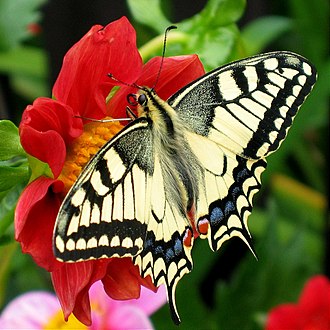
The study found that different host plants with similar butterfly assemblages produce chemical defences with similar composition. Meanwhile phylogenetic relatedness did not result in similarities between butterfly assemblages. These observations were limited to only the Pieridae and Lycaenidae networks. The findings of the study are significant. This because it does refute the theory that higher plant diversity correlates with higher herbivore diversity (Linden et al., 2021).
Similar patterns have been observed that in European Grassland Systems. Plant phylogenetic alpha diversity poorly predicts butterfly phylogenetic alpha diversity (Pellissier et al., 2013). Phylogenetic measures of plant diversity do not represent all the functional space available within an herbivore community. Host plant chemical production, amongst other defensive strategies are better predictors (Pellissier et al., 2013).
Factors influencing Coevolution
As such, there are other factors which influence the coevolutionary patterns on interacting species. This includes variation in the composition of interacting communities (Thompson, 1999). For example, the presence of other short-tubed flowers as nectar sources restricts the coevolutionary process between P. ganglebaueri and Z. microsiphon. On the contrary, in simpler communities which lack alternative short-tubed nectar plants, escalatory coevolution between the fly and (Z. microsiphon) have been observed (Anderson and Johnson, 2007).
While coevolution does to a great extent influence the evolution of morphological traits, it is often in collaboration with abiotic factors. (Anderson and Johnson, 2007). For example, despite the significance of coevolutionary pressures, regression methods suggests that altitude and fly thorax width also induce. sequential trait evolution in fly proboscis length (Jermy, 1976). Specifically, lower temperature and strong winds may constrain the evolution of fly proboscises length at higher altitude. (Anderson and Johnson, 2007).
Coevolution of Plants and Mammalian Herbivores
Furthermore, it must be noted that the coevolution of herbivore and their host plants is not restricted to insect-plant interactions. Extensive coevolutionary patterns have been observed amongst mammalian ungulates and grasses. It has been suggested that hypsodont evolved in grazers as a response to the appearance of open grassland habitats (Strömberg et al., 2013).
The possession of high crowned teeth and an extended enamel allows grazers to consume the phytoliths, silica rich granules associated with grasses, without significant wear and tear. It is for this reason that it has been hypothesises that hypsodont evolved in ungulates concurrently with the spread of grasslands (Strömberg et al., 2013).
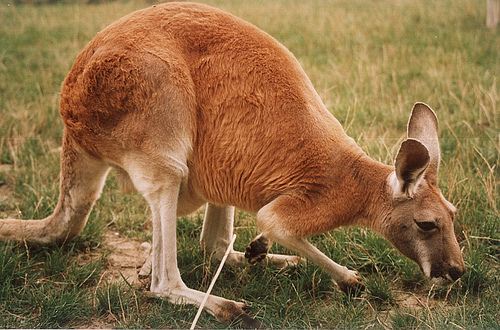
As such, fungal endophytes are often utilized by plants to deter ungulate herbivory through the production of toxic alkaloids (Tanentzap et al., 2014). However, some species, including moose (Alces alces) and European reindeer (Rangifer tarandus) have evolved to counter the defences produced by such grass–endophyte mutualism. It was observed that the saliva of both species was able to inhibit the production of ergot alkaloids by foliar endophyte (Epichloë festucae) in red fescue (Festuca rubra). (Tanentzap et al., 2014). No wonder grasses are a big portion of biodiversity.
Conclusion
However, as mentioned earlier, the role of coevolution in the diversification of mammalian herbivores is not as pronounced compared to that observed in insect herbivores. Nonetheless, this distinctly highlights the role of coevolution in the diversification of species. Couple with abiotic variations, coevolution certainly is a major force of evolution. Furthermore, considering the intricate relationships between plants and herbivores, it is not surprising that species are changing their behavior significantly due to climate change.