In recent decades, human activities have caused severe ecological impacts, such as habitat degradation, soil erosion and loss of biodiversity. While the biodiversity crisis can solely be attributed to anthropogenic causes, human activity also significantly impacts the evolution of non-human animals (Carroll et al., 2007). In fact, anthropogenic activities are the underlying cause of most examples of contemporary evolution. This refers to evolution of organisms occurring over ecological time scales (Carroll et al., 2007). The importance of human activities on the evolution of non-human animals can be further understood through two distinct frameworks. These are the phenotypic adaptive landscape and the eco-evolutionary framework. These are visually represented by figure 2 (Hendry, Gotanda and Svensson, 2017).
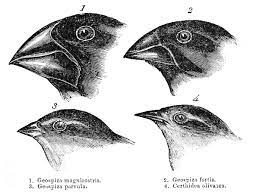
What are Phenotypic Adaptive Landscapes?
Phenotypic adaptive landscapes refer to multidimensional surfaces that associate the mean population fitness with mean phenotypic values of the population. Valleys of the surface illustrate low fitness phenotypes while peaks are representative of high fitness phenotypes (Hendry, Gotanda and Svensson, 2017). Natural selections favours phenotypes which are in proximity to these adaptive peaks. Human activities modify these landscapes either by altering the adaptive peak or the distribution of phenotypes (Hendry, Gotanda and Svensson, 2017). Aspects of the adaptive peak, such as gradient and elevation, can be altered by strong directional, disruptive and divergent selection. Comparatively, phenotypic distribution is influenced by changes in gene flow, plasticity and hybridization within a population. (Hendry, Gotanda and Svensson, 2017).
What is the eco-evolutionary framework?
The eco-evolutionary framework attempts to understand the broader implications of human induced changes in the phenotypic adaptive landscape at a population, community and ecosystem level (Hendry, Gotanda and Svensson, 2017). The subsequent changes in ecological conditions eco- feedbacks to the human societies, resulting in changes in the disturbance patterns. On the contrary, the broader, ecological effects of human activities have an indirect evolutionary consequences on individual populations, which also eventually influences human activity patterns (Hendry, Gotanda and Svensson, 2017). This results in a cycle of interactions between human disturbances and the eco- evolutionary framework, indicating their intricate interdependence. It is this cycle which highlights the importance of various human activity, both directly and indirectly, on the evolution of non-human animals (Hendry, Gotanda and Svensson, 2017).
Evolution through Directional Selection
One of the most prominent mechanisms through which anthropogenic activities propagate evolution in non-human species is by exerting directional selection on an extreme phenotype. This results in the shift of allele frequency towards the direction of the particular phenotype (Hendry, Gotanda and Svensson, 2017). Amongst anthropogenic disturbances, selective harvesting, in the form of trophy hunting and fisheries, exert the strongest directional selection (Hendry, Gotanda and Svensson, 2017).
Individuals with desirable traits, such as large body size or large horns, are disproportionately removed from the population by harvesters (Hendry, Gotanda and Svensson, 2017). As a result, the population will experience directional selection against the desirable traits (Traill, Schindler and Coulson, 2014). The harvested population will probably experience a loss of fitness as the desired trait is usually an adaptive peak (Hendry, Gotanda and Svensson, 2017).
Directional selection of traits by harvesting has been observed in numerous species. For example, illegal poaching for ivory tusks has led to the increased prevalence of tusklessness in numerous populations of African savannah elephants (Loxodonta africana). This observation can be attested to by a whole genomic study. This study found a substantial increase in X-linked dominant, lethal inheritance of tusklessness in females in Gorongosa National Park, Zambia, following severe bouts of ivory poaching (Campbell-Staton et al., 2021).
Opposing the Adaptive Peak
Generally, as the desirable trait is the adaptive peak, harvesting imposes a strong directional selection opposite to that produced by natural selection (Traill, Schindler and Coulson, 2014). For example, in natural environments there is a strong selection for larger body sizes for fish, as this is associated with increased fecundity and predator survival. In contrast, larger fish, which are more profitable for fisheries, have a higher mortality rate in harvested population than their smaller counterparts who are able to escape the fishing mesh. Therefore, fisheries cause directional selection towards smaller fish which reach sexual maturation earlier (Traill, Schindler and Coulson, 2014).
Despite acting in opposing directions, selection pressures due to natural mortality and harvesting, rarely nullify due to a mismatch in the rate of phenotypic change (Stenseth and Dunlop, 2009). In fact, a metanalysis found that the rate of phenotypic change in populations exposed to hunting and other anthropogenic disturbances were 300% and 50% greater than populations exposed to only natural selection respectively (Stenseth and Dunlop, 2009).
From this, it can be inferred that harvesting causes significantly stronger directional selection than that produced by natural mortality. This can be attributed to the significantly greater mortality rates associated with harvested populations. For example, commercial fisheries have a mortality rate which is 400% greater than the natural rates. Consequently, fisheries have a significantly higher turnover rate and rate of phenotypic change (Stenseth and Dunlop, 2009).
Not the case with Bighorn Sheep!
However, it has been argued the phenotypic changes due to human activities are not always evolutionary responses as observed in populations of trophy hunted bighorn sheep (Ovis canadensis) (Traill, Schindler and Coulson, 2014). The relative reduction in body mass when exposed to increased harvest is restricted to only adult rams. If trophy hunting did indeed incite an evolutionary response, increased harvest would have also caused a relative reduction in body mass in adult ewes.
The reduction of mean body weight in rams, therefore, can be attributed to the harvesting of heavier individuals from the population, rather than directional selection (Traill, Schindler and Coulson, 2014). This suggests that a reduction in body weight and horn size in harvested was a demographic response to trophy hunting rather than evolutionary (Traill, Schindler and Coulson, 2014).
Evolution through Diversification
Anthropogenic disturbances also influence the evolution of non-human animals through evolutionary diversification (Hendry, Gotanda and Svensson, 2017). This occurs when species develop adaptations to either a separate peak on a single adaptive landscape or to a similar peak on a different landscape (Hendry, Taylor and Donald McPhail, 2002). Once exposed to modified environments or novel locations, a species experiences varied degrees of gene flow, hybridization, disruptive and divergent selection. An example of this is a change in altitude habitated by butterflies.
These result in evolutionary diversification (Hendry, Taylor and Donald McPhail, 2002). Humans can facilitate evolutionary diversification through the introduction of non-native species and creation of novel habitat (Hendry, Gotanda and Svensson, 2017).
The introduction of non-native species to an ecosystem is an example of evolutionary diversification due to exposure to a novel adaptive landscape. (Hendry, Gotanda and Svensson, 2017). Selection factors associated with the novel location, coupled with the lack of gene flow from the source population, encourage the emergence of novel adaptations and genetic divergence respectively (Hendry, Gotanda and Svensson, 2017).
Moreover, biotic interactions also assist the diversification of population. For example, the homoploid hybrid speciation of tephritid fruitflies R. mendax and R. zephyria was catalysed by the simultaneous infestation of the non-native brushy honeysuckle forms Lonicera spp. as the host by both species (Schwarz et al., 2005).
The role of Urban Landscapes
Evolutionary diversification can also be effectuated through the exposure of novel selection pressures associated with unfamiliar habitats, such as urban landscapes (Bull and Maron, 2016). While urbanization is associated with extensive biodiversity loss, few species are able to exploit and colonize these foreign environmental conditions (Mueller et al., 2013). This is through the emergence of novel adaptations to counter the unfamiliar selection pressures (Mueller et al., 2013). For example, urban population of Eurasian Blackbird (Turdus merula) display increased neophiliac behaviour, which allows for greater exploitation of novel resources in urban landscapes (Mueller et al., 2013). Additionally, urban populations have shortened circadian rhythms due to the prevalence of artificial lighting (Mueller et al., 2013).
Urbanization also promotes evolution by limiting gene flow between urban and rural populations. For example, urban blackbirds display reduced migratory behaviour compared to that of their rural counterparts. Not only does this reduce the genetic flow between urban and rural population but also promote earlier breeding and fostering fosters genetic divergence (Evans et al., 2011).
Habitat Fragmentation induces Divergent Selection
Habitat fragmentation due to economic development also facilitates divergent selection. An example of this is the divergence of the London underground mosquito (Culex moletus) from the common house mosquito (Culex pipiens) following the latter’s colonization of the London underground. Habitat fragmentation created a barrier to gene flow. The reproduction isolation of the surface and subterranean populations, resulted in the accumulation of genetic variation and eventual speciation (Byrne and Nichols, 1999).Arguments have been made that the reduction in genetic diversity of urban populations is due to founder effects and genetic drift rather than genetic adaptations.
Anthropogenic activities also have a significant impact on the evolutionary potential of a species. This is by influencing the genetic variation of its populations. Greater genetic variation allows for increased adaptiveness to changing environments. The translocation of species into non-native habitats and exposure to pollution and mutagens influences genetic variation. A quantative literature review found that habitat fragmentation is known to significantly reduce genetic variance (DiBattista,2007). Pollution, meanwhile reduced genetic variation non significantly. Harvesting did not have any influence on genetic variation (DiBattista, 2007). Changes in neutral genetic variation due to human activities were weak but consistent within natural populations. (DiBattista, 2007)
Conclusion
There is more than sufficient evidence correlating anthropogenic activities with the contemporary evolution of non-human animals. However, it is highly unlikely that human activities have resulted in a substantial increase in the rate of contemporary evolution (Carroll et al., 2007). Genetic change due to human mediated contemporary evolution remains modest despite the extensive magnitude of human disturbance across ecosystems (Carroll et al., 2007). In reality, the observed rates of contemporary evolution have probably remained stable throughout history, uninfluenced by the onset of the Anthropocene (Carroll et al., 2007).
To conclude, contemporary evolution of non- human animals is amongst the probably amongst the most prominent consequences of anthropogenic activities and disturbances. Human induced evolution utilizes numerous mechanisms, such as evolutionary diversification, directional selection, changes in genetic variation and loss of gene flow.
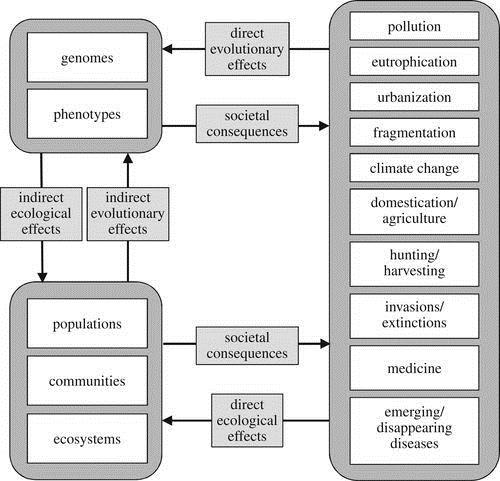
Help us Help Them! Think Wildlife Foundation is a non profit organization with various conservation initiatives. Our most prominent campaign is our Caring for Pari intiative. Pari is a rehabilitated elephant at the Wildlife SoS Hospital. 25% of the profits from our store are donated to the elephant hospital for Pari. Other than buying our wonderful merchandise, you could donate directly to our Caring For Pari fundraiser.