Interactions between flora and fauna occurs in three primary forms, namely pollination, herbivory and parasitism. Extensive chemical communication facilitated these interactions. Plants produce volatile organic compounds. These primarily function to either attract pollinators or provide protection against to herbivores. In response, numerous modes of chemical communication have evolved in fauna species. This is in effort to derive the maximum fitness benefit through interactions with their plant hosts. Chemicals certainly have had a role to play in the evolutionary arms race between plants and herbivores!
Volatile Organic Compounds
Chemical communication is extensively used as offensive and defensive mechanisms by herbivores and plants respectively. Herbivores utilize volatile organic compounds for the detection of their respective host plant. A study found that odour intensity (VOC emmisions) positively correlated with the rate of discovery of the leaves of Eucalyptus punctata by swamp wallabies (Wallabia bicolor). In fact, when the plant odour was completely suppressed, wallabies took significantly longer to detect visually obscured plants at night.
Similarly, spider monkeys (Ateles geoffroyi) displayed greater utilization of olfaction to identify edible fruit from their non edible counterparts when visual cues proved insufficient for the evaluation of fruit quality (Finnerty et al., 2017). This highlights the significance of olfaction in mammalian foraging, particularly in the absences of visual cues. (Finnerty et al., 2017). However, the further studies are required to truly understand the role of chemical communication in mammals, particularly while foraginDefense against Herbivores
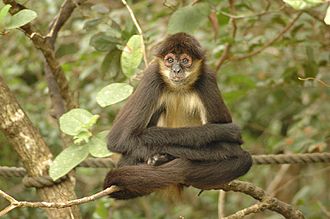
Plants rely on Chemical Defenses
Toxins
Herbivory has significant fitness costs as it risks the survival and the reproductive success of plants. For this reason, the evolution of robust defensive strategies is imperative. Floral chemical cues are crucial defense against herbivory. Chemical refences broadly consist of terpenes, phenolics, alkaloids and cyanogen. These toxins cause significant physiological damage to herbivores. For example, the consumption of terpenes can inhibit adenosine triphosphate (ATP) formation in insects (Langenheim, 1994). Another example includes the emission of (z)-3- hexenylvicianoside by larvae infested tomatoes. Upon transmission, these compounds convert into glycoside. Subsequently the growth of insect larvae is restricted (Langenheim, 1994).
HIPV
Chemical defences, such as Herbivore Induced Plant Volatiles, also protect plants from herbivory through an indirect mechanism. This is through the attraction of the predators. For example, a tobacco species (Nictonia attenuate) produces branched chain aliphatic acids through its O-acyl sugars (Weinhold and Baldwin, 2011). These compounds attract omnivorous ants. This species predate on Manduca sexta larvae, one of the primary herbivores of the plant (Linden et al., 2021).
Another example includes the alteration of volatile chemical composition of apple trees (Malus silvestris ) when infested with the winter moth (Operophtera brumata). As depicted in Figure 1, the volatiles of infested trees volatiles possessed increased quantities of α-farnesene and dodecanal and less 3-octanol and 1-none compared to the volatiles of trees lacking larvae. Due to these differences, Great Tits (Parus major) were able to detect infested trees and predate on the winter moth larvae (Amo et al., 2013).
Resistance to The Plant Chemical Defences
Unfortunately for plants, numerous herbivores have developed a resistance to volatile organic chemicals. For example, the Corn Earworm caterpillar (Helicoverpa zea) secretes glucose oxidase on leaves. Subsequently, this causes stomatal closure in soybean (Glycine max) and tomato (Solanum lycopersicum). As a result, this inhibits the emission of several HIPVs, including (Z)-3-hexenol, (Z)-jasmone and (Z)-3-hexenyl acetate. Furthermore, herbivorous insects possess enzymes which detoxify plant chemical defenses. This is through oxidation, reduction, hydrolysis or molecular conjecutation (Mithöfer and Boland, 2012).
A prominent example is the diversification of P450 monooxygenases in the black swallowtail (Papillio polyxenes). P450 monooxygenases promotes detoxification of the plant toxin furanocoumarins. This therefore, allows for the consumption of the plant (Mithöfer and Boland, 2012). Similarly, the saliva of moose (Alces alces) and European reindeer (Rangifer tarandus) have evolved to inhibit the production of ergot alkaloids by foliar endophyte (Epichloë festucae) in red fescue (Festuca rubra).The lack of this toxin allowed for the efficient digestion of the red fescue (Tanentzap et al., 2014). The resistance of herbivores to these volatile chemicals forces their host plant to continue evolving their chemical defenses.
Chemical Communication has fostered an Evolutionary Arms Race
The evolutionary arms race between the offensive and defensive mechanisms in a herbivore- plant system was highlighted by a phylogenetic study of volatile chemistry of the genus Bursera. The study found a strong positive evolutionary trend with increasing chemical diversity and complexity (Becerra, 1997). Younger species possessed a more elaborate suite of secondary metabolites. This suggests the evolution of a comprehensive chemical defensive strategy (Becerra, 1997).
Such accumulation of VOCs can be attributed to the conflicting information theory. Herbivores can identify host plants through the detection of a only few VOCs. By increasing the diversity of VOCs in their chemical profiles, plants can circumvent detection by their respective herbivores (Zu et al., 2020). This “escape and radiation” model has been observed in butterfly assemblages.
A study found that different host species sharing similar Lycaenidae and Pieridae butterfly assemblages possessed volatile emissions with similar compositions (Linden et al., 2021). This highlights the possible role of plant chemical defences in a broader community perspective. By producing apparently redundant volatile chemicals, which are shared by other species, a particular plant can complicate the host identification process for herbivores (Zu et al., 2020).
Chemical Communication with Parastoids
HIPVs also negate herbivory pressure by attracting parasitoids. These are organisms, consisting predominantly of wasps, that live in close association with a host. Eventually parasitoids cause the death of its host. As such, parasitoids have evolved highly specialized responses to plant volatiles emitted in defense against herbivorous insects.
For example, Cotesia glomerata wasps are attracted to the volatile emissions of black mustard (Brassica nigra) infested with Large Cabbage White Butterfly (Pieris brassicae). Infested plants produced greater emissions of green-leaf volatile (Z)-3-hexen-1-ol. The wasps reduce herbivory pressure by parasitizing the larvae (Hu et al., 2020).
Chemical Defences against Parasitoids
As such, significant variations were observed in the volatile profiles of plants infested by N. lugens and C. suppressalis separately (Hu et al., 2020). However, no such variations were observed in volatile profiles of plants individually infested by C. suppressalis. Similar observations were noted with plants infested by both species. This highlights the possibility that N. lugens induced volatiles were masked by those of C. suppressalis (Hu et al., 2020). Such strategies to avoid parasitoids are not limited to this scenario.

For example, predatory mites are less attracted to spider mite infested lime bean plants in the presence of whiteflies . This can be attributed to the reduced emission of (E)-β-ocimene (Hu et al., 2020).
Hyperparsitoids
As such, the role of chemical communication in herbivore-plant ecology is further complicated by hyperparasitoids. These organisms, utilize HIPVs to detect, and eventually parasitize, primary parasitoids. For example, the hyperparasitoid Lysibia nana displayed greater attraction towards the volatiles of brassicaceous plant infested by two primary parasitoids, Cotesia rubescula and C. glomerata, over that of undamaged plants. (Poelman et al., 2012)
HIPVs used in Predator and Prey Interactions!
The HIPVS induced by a particular herbivore is often utilized by other herbivores to escape detection by natural predators (Hu et al., 2020). For example, brown planthopper (Nilaparvata lugens) display preferential oviposition on rice plants infested by rice striped stem borer (Chilo suppressalis) (Hu et al., 2020).
Striped stem borer infested plants are generally avoided by Anagru nilaparvatae, an egg parasitoid which is the primary predator of Nilaparvata lugens (Hu et al., 2020). This avoidance is attributed to the presence of chemical repellents, such as (E)−2-heptenyl acetate, 2-nonanone, 2- tridecanone, 2-heptanol, D-limonene and α-pinene, which are characteristic of the volatile profiles of C. suppressalis infested plants (Hu et al., 2020).
Herbivorous insects are also known to utilize secondary plant metabolites as resistance towards their predators (Hu et al., 2020). For example, woolly bear caterpillar (Grammia geneura) feed on relatively toxic host plants, such as Senecio longilobus and Ambrosia confertiflora, both of which contain pyrrolizidine alkaloids. The consumption of these toxic plants, provides the caterpillar defence against the parasitic common tachinid fly (Exorista mella) (Hu et al., 2020).
Pollination depends on Chemical Communication
It must be noted, however, that chemical communication between plants and animals is not limited to agonistic interactions. Mutualistic interactions between flora and fauna, also exhibit elaborate chemical communication. This is not surprising, given that animals, particularly insects, areresponsible for the pollination of 88% of angiosperms. Generally, pollen attaches to the insect either during oviposition or the consumption of nectar. Furthermore, the diversification of angiosperms, has often been credited accredited to animal pollination. Numerous pre and post pollination barriers ranging from geographical isolation, morphological incompatibilities, pollen competition and hybrid sterility have resulted in angiosperm speciation. Pollinators, however, have a significant role in the evolution of angiosperms.
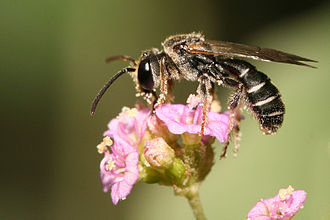
Pollination has diversified Angiosperms
The diversity of angiosperms can be to a great extent attributed to pollinator specificity toward plant signals. For example, the presence of a single volatile compound (indole) in the flower Impomopsis tenuituba is able to attract hawkmoths. Meanwhile, the species is not attracted to the flower’s close relative, Ipomopsis aggregate, which lacks this volatile compound. It must be mentioned however, that hawkmoths only fed on white I. tenuituba flowers. This emphasizes the combined role of both olfactory and visual signals in attracting the respectective pollinators.
Such high levels of host specificity have been observed between figs (Moraceae) and fig wasps (Agaoniae) mutualism. As such, scent is the primary signal involved in pollinator attraction in these mutualistic relations. A study found that most fig wasps were only attracted to the volatile chemicals of their specific host (Souto-Vilarós et al., 2018).
It was surprisingly to note that even small divergence in scent composition between closely related Ficus species promoted significant reproductive isolated though pollinator behaviour. This emphasizes the role of pollinator specificity towards host volatile compounds in the diversification of angiosperms. (Souto-Vilarós et al., 2018). It must be noted, however, that chemical communication between pollinator and host is not limited to such interactions.
Pollination through Carrion Mimicry
Another prominent form of pollination which relies on chemical communication between insects and plants is mimicry. A common example where mimicry is used for pollination purposes is in carrion flowers. These species mimic both, the smell and the appearance, of rotten animal carrion.
The gas chromatography analysis found that the composition volatile compounds of carrion flowers resemble that of decomposing carrions (Johnson, 2016). The compounds consist of oligosulfides which originate from protein degradation. These volatile compounds attract a variety of insects, such as flesh flies (dacophagidae), blowflies (calliphoridae), house flies (muscidae) and some beetles, including Dermestidae and Silphidae. The antennae of these flies and beetles respond to the dimethyl disulfide and dimethyl trisulfide hence causing an attraction towards the flower (Johnson, 2016).
The mimicry of the carrion flowers results in these insects ovipositing on the flowers ,which results in the eventual transfer of pollen. As such, oviposition on non-flower substrates is fairly costly for insects. However, carrion flowers are rare. For this reason, the fitness costs of an individual duped insects do not significantly hinder the fitness of the entire species (Johnson, 2016). Another example of mimicry is by the angiosperm Certaocaryum argeneum. The large seeds of this plant resemble the faeces of the bontebok. In fact, it contains chemicals, such as acetophenone, phenol, p-cresol and 4-ethylphenol, which are all typical of herbivore faeces. The beetle Epirinus flagellates end up falsely ovipositing, hence resulting in the pollination of the angiosperm
.